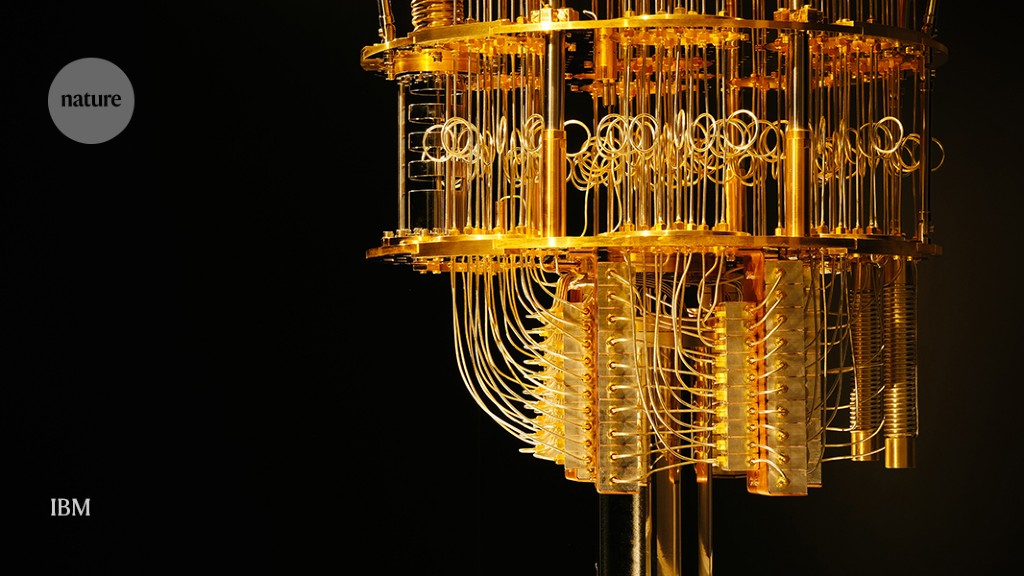
The underdog technologies are gaining ground in the quantum computing race
Quantum Materials for Consumer Electronics: The Rise and Fall of Molecules in the Age of Quantum Mechanics
Technologies enabled by quantum science will help researchers better understand the natural world and harness quantum phenomena to benefit society. They will transform health care, transportation, and communications, and enhance resilience to cyber threats and climate catastrophes. For example, quantum magnetic field sensors will enable functional brain imaging; quantum optical communications will permit encrypted communications; and quantum computers will facilitate the discovery of next-generation materials for photovoltaics and medicines.
Qubits, the building blocks of quantum computers, rely on materials with quantum properties, like electron spin, which can be manipulated. Once we can harness these properties, we can control them using light and magnetic fields, creating quantum phenomena such as entanglement and superposition. There are Josephson junctions that can conduct electricity at super-low temperatures and they are called superconducting qubits. The harsh temperature and high-frequency operation requirements mean that even the most basic aspects of these superconducting qubits—the dielectrics—are tricky to design. The qubits themselves have to be small to store electrical field energy, and crosstalk between adjacent qubits makes the noise worse. It’s not feasible to get to the millions of qubits required for a quantum computer with these materials.
The design of materials for quantum technology will be more innovation in the next few years. There are many awesome candidates considered so far, but the use ofmolecules is one that I am most excited about. These materials are designed around carbon-based organic semiconductors, which are an established class of materials for the scalable manufacture of consumer electronics (having revolutionized the multibillion-dollar OLED display industry). We can use chemistry to control their electronic properties, and the infrastructure surrounding their development depends on expert knowledge.
For example, chiral molecular materials—molecules that exist as a pair of non-superimposable mirror images—will revolutionize quantum technologies. Thin, single-handed layers of these remarkably versatile molecules can be used to control the spin of electrons at room temperature. At the same time, the long spin coherence times and good thermal and chemical stability of metal phthalocyanines will see them being used to carry quantum information.
The pace of improvement in neutral atoms has surprised the quantum-computing community. The path to scale to a thousand of atomic qubits is clear and will likely happen within two years, according to physicist Chao-Yang Lu.
The technique, which was barely on the radar, is going to break the 1,000-qubit barrier soon. It traps neutral atoms using tightly focused laser beams, called optical tweezers, and encodes qubits in the electronic states of the atoms or in the spins of atoms’ nuclei (see ‘Laser tweezers’). Giulia Semeghini is a physicist at Harvard University and she says that the approach has been growing for over a decade.
Google made headlines in 2019 when it claimed that a machine made of 54 superconducting qubits had performed the first quantum computation that would have taken impossibly long on a classical computer, an achievement that researchers call quantum advantage. IBM will be the first company to produce a 1,000-qubit quantum chip, which it expects to unveil in the next few months.
Physicists split a single laser beam into many in order to assemble multiple qubits, by using a screen made of liquid crystals. A lot of tweezers can be created, each trapping their own atom. The atoms are typically a few micrometres away from their neighbours, where they can persist in a quantum state for several seconds or more. To make the atoms interact, physicists point a separate laser at one of them to tickle it into an excited state, in which an outer electron orbits much farther away from the nucleus than normal. This boosts the atom’s electrostatic interactions with a neighbour.
One major advantage of the technique is that physicists can combine multiple types of tweezers, some of which can move around quickly — with the atoms they carry. “Every time you want two of them to interact, you bring them together,” says Harvard physicist Dolev Bluvstein. It makes the technique more flexible than other platforms in which qubit can only communicate with one another on the chip. In April 2022, the team including Bluvstein and Semeghini demonstrated their flexibility.
A team led by physicists at the University of New South Wales in Australia assembles chips using the tip of an atom, and this could be used to make chips similar to current computer chips. “Everything is patterned with sub-nanometre precision,” she says.
Yet another approach is still at the conceptual stage, but it has received substantial investment, by Microsoft in particular. The technique tries to exploit topological states to make qubits robust to degradation, similar to a knot that can be twisted and pulled but not untied. The basic mechanism for one kind of protection was observed in 2020 and now researchers are working on demonstrating the first qubits.